HISTORY
The Dual Nature of Light: From Descartes to Carbone
- Published On
September 27th, 2024
- Author
Hassan Muzzafar Haq
In 1600, René Descartes said that light is a wave and demonstrated this by putting a coin in a mug of water, which showed reflection and refraction. Without water, there was no such phenomenon. In one of his experiments, he produced a rainbow by using a water-filled glass sphere and sunlight. He explained that the refraction of light caused the formation of rainbows. He then studied the refraction and emergence of colors of light in a prism. He observed that the different colors of light refracted at varying degrees. René Descartes became convinced that light was essentially a wave, one that moved through a mysterious substance called plenum.
Early Stages
In 1670, Newton, a brilliant physicist and mathematician who changed our understanding of the universe with his laws of motion, said that light is a particle because only particles can describe motion in a straight line. His idea was so strong that it gave much weight to science. Isaac Newton thought light was a particle, but he was at a loss for a way to explain many of its properties, like the way it refracted and split in a prism. Newton's model of light proposes that the propagation of light is caused by the rectilinear motion of light particles, which he termed as corpuscles. These light particles travel at a finite speed, and their interactions with the external environment, e.g., rigid surfaces, walls, and the human eye, obey Newtonian physics. Light exhibited frequency-like properties when he used a prism to split sunlight into its component colors. He argued that light does not exhibit diffraction: ‘We can hear sound from behind an obstacle, but we don’t see light.’
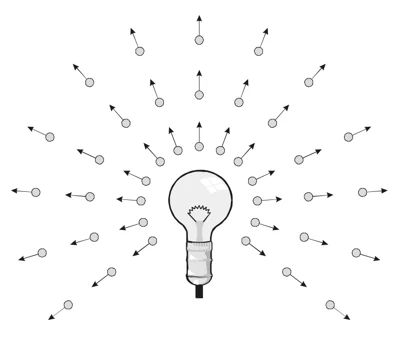
Newton's model of light suggests that light travels in straight lines due to the movement of tiny particles he called light corpuscles.. (Photo Credit: Science Ready Australia)
At the same time, Huygens showed that light reflects, refracts, and diffracts. In 1678, Huygens proposed a model where each point on a wavefront may be regarded as a source of waves expanding from that point. The expanding waves may be demonstrated in a ripple tank by sending plane waves toward a barrier with a small opening.
Youngs's Double Slit Experiment
In 1801, Thomas Young’s double-slit experiment used two coherent sources of light placed at a small distance apart. Usually, only a few orders of magnitude greater than the wavelength of light are used. Young’s double-slit experiment helped in understanding the wave theory of light. He did this with electrons as well. When electrons strike slits, they behave as waves. This leads to the analogy that either light is a particle behaving as a wave, or an electron is a wave behaving as a particle.
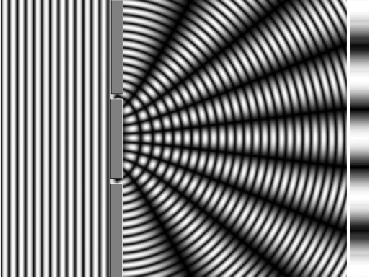
Young's slit experiment demonstrates that when light passes through a pair of vertical slits, it creates a diffraction pattern on a screen, showing numerous vertical lines spread out horizontally. (Photo Credit: Medium)
In 1818, Augustos Fresnel He came up with the idea that if you have a beam of light and you put some obstacle, a circular piece in between, depending on how light behaves going around the circular piece, showing it’s a wave or a particle. If light were a wave, it diffracts around the edge of the obstacle and produces a spot in the center of the shadow. If it’s a particle, it produces a perfect shadow of the obstacle. His result is considered absurd because we could not see it in everyday life. François Arago insisted that this experiment has to be done with monochromatic light. The dot shows that light is a wave, named as Poisson’s dot.
Maxwell's Theory Of EM Waves
In 1864, James Clark Maxwell performed an experiment; changing an electric field produces a time and space-varying magnetic field, and a changing magnetic field produces a time and space-varying electric field. The changing electric and magnetic fields result in the propagation of electromagnetic waves (or light waves) even in a vacuum. considered as coming in packets instead of waves. Planck named these packets quanta, that is, radiation consists of specific energies determined by a new fundamental constant, thereafter called Planck's constant. But it is somewhere incomplete in basic assumptions.
Heinrich Hertz’s experiment
Finally, in 1888, the German physicist Heinrich Hertz showed that EM waves indeed exist and behave exactly as Maxwell had predicted. Hertz generated the waves by applying an alternating current to an air gap between two metal balls. The width of the gap was such that a spark occurred each time the current reached a peak. A wire loop with a small gap was the detector; EM waves set up oscillations in the loop that produced sparks in the gap. Hertz determined the wavelength and speed of the waves he generated, showed that they have both electric and magnetic components, and found that they could be reflected, refracted, and diffracted, showing light as a wave.
Max Planck's Theory
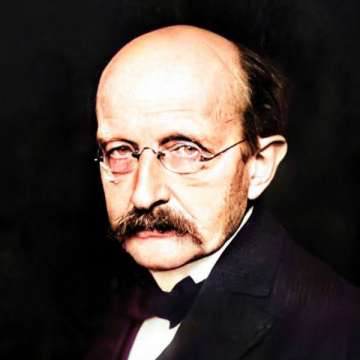
His theory states that energy can only be transferred in quantized amounts, and that the energy of the radiation absorbed or emitted is directly proportional to the frequency of the radiation (Photo Credit: Geniuses)
Max Planck studied discrete energy from particles, showing light is a particle. Max Planck solved this problem in 1900 by introducing the theory of “quanta”, light emitted and absorbed by the hollow black sphere had to be considered as coming in packets instead of waves. Planck named these packets quanta, that is, radiation consists of specific energies determined by a new fundamental constant, thereafter called Planck's constant. But it is somewhere incomplete in basic assumptions.
Einstein’s Photoelectric Effect
In 1905, Einstein came along using Planck's model and explained the photoelectric effect, where light on the metal surface gives off electrons. Light, Einstein said, is a beam of particles whose energies are related to their frequencies according to Planck's formula. When that beam is directed at a metal, the photons collide with the atoms. If a photon's frequency is sufficient to knock off an electron, the collision produces the photoelectric effect.
The more light, the more electrons, and the higher the frequency, the higher the energy of emitted electrons. A little bit of high-frequency light gives a little bit of high-energy electrons. And vice versa. Here Einstein says perhaps it is not a particle. Perhaps it is sometimes a particle. Further, he says if light is present in several chunks of energy particles, (the particle of light) then you can measure its momentum of these particles.
In 1923, Arthur Compton measured the momentum of particles experimentally. The aim of this experiment is to look at how the scattering angle is related to photon energy in Compton Scattering. The Compton effect describes the increase in the wavelength of photons (X-rays or gamma rays) as a result of scattering by a charged particle (usually an electron). During the study, Compton found that the wavelength is not dependent on the intensity of incident radiation.
In 1924, Louis de Broglie introduced the idea that particles, such as electrons, could be described not only as particles but also as waves. This was substantiated by the way streams of electrons were reflected against crystals and spread through thin metal foils.
This all happens against the backdrop of quantum physics, so Heisenberg, in 1926, acknowledged that the more precisely you know the position of a particle, the less precisely you will know about its momentum, and vice versa. It states that it's not possible to simultaneously measure both the position and momentum of a microscopic particle. Mathematically, it is given as: Δx × Δp ≥ 4πh.
Max Born
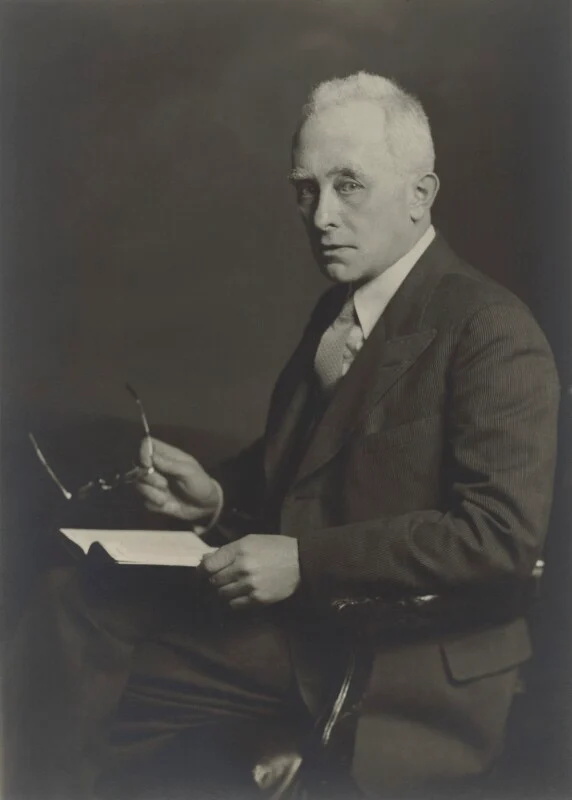
A portrait of Max Born, a German-British physicist key to developing quantum mechanics, with contributions to solid-state physics and optics, and mentor to notable physicists in the 1920s and 1930s. (Photo Credit: National Portrait Gallery)
Max Born says the nature of assets of a particle is governed by probability but not by any property of the particle itself. He says the probability density of finding the particle at a given point is proportional to the square of the magnitude of the particle's wave function at that point. Einstein hated this saying of Max Born, and his famous quote came from the same, "I, at any rate, am convinced that He GOD does not play dice."
The previous century, in 1900, all theories convinced that light was a wave, but in the 20th century, all were coming towards its particle nature.
The Fifth Solvay Conference in 1927 gathered the greatest minds of the 20th century to discuss the nature of the electron and photon. The leading figures were Albert Einstein and Niels Bohr. Seventeen of the 29 attendees were or became Nobel Prize winners, including Marie Curie, who, alone among them, had won Nobel Prizes in two separate scientific disciplines.
Wave vs. Particle in Different Experiments
Bohr regarded the "duality paradox" as a fundamental or metaphysical fact of nature. A given kind of quantum object will exhibit sometimes wave, sometimes particle, character in respectively different physical settings. He saw such duality as one aspect of the concept of complementarity.
Neil Bohr's controversial idea was discarded later, but the equation that describes light as a wave describes where you could find the particle of light; it didn’t exist until you looked for it. The very fact of observing the light causes the photons to exist. This tells us that photons do not have any independent reality. He says the same for electrons; electrons only exist as particles when we observe them; otherwise, they are waves obeying double-slit.
Light Simultaneously Exhibits Wave and Particle Properties
Fabrizio Carbone, a physicist in Switzerland, and his collaborators beamed photons into a 40-nanometer silver wire and observed what happened to a stream of electrons running along the surface of the wire. As the electrons traveled along the photon-filled wire, they were influenced by the photons directly and indirectly. When a photon hit an electron, it transferred some energy, speeding up or slowing down the electron depending on the angle of the strike. The electrons were also excited by the light’s wave-like nature, though not through direct contact.
Subscribe To Our Newsletter
Receive amazing space news and stories that are hot off the press and ready to be read by thousands of people all around the world.