PLANETS
Exoplanets And The Search For Earthlike Worlds
- Published On
November 12th, 2024
- Author
Syed Muhammad Hassan, Mustafa Ali
Table Of Contents
- Published On
November 12th, 2024
- Author
Syed Muhammad Hassan, Mustafa Ali
Introduction
Silence—a heavy, intrusive presence that fills the gaps between thoughts, unsettling the mind. It’s the kind of silence that envelops you when you stand on the brink of something vast, uncharted, and cold. Humanity has always been uneasy with silence. In its stillness, our fears and uncertainties grow louder, driving us to break it. This relentless urge to fill the void has extended beyond Earth, reaching into the cosmos. Our exploration of space is not just a quest for knowledge; it’s a desperate search for a world where life can thrive, a refuge from cosmic solitude. Humanity's venture into the great unknown is as much about discovery as it is a battle against isolation.
Exoplanets And Their First Discovery
In 1992, this cosmic silence was softly interrupted by a groundbreaking discovery. Astronomers Aleksander Wolszczan and Dale Frail identified two planets orbiting a pulsar, PSR B1257+12, located 2,300 light-years away in the constellation Virgo. Pulsars, a type of neutron star, emit incredibly regular radio pulses, which in this case showed slight irregularities at consistent intervals. After thorough analysis, Wolszczan and Frail concluded these irregularities were caused by two planets, three and four times the mass of Earth, orbiting the pulsar and rotating every 67 and 98 days. These pulsar planets eventually came to be known as exoplanets, which by definition are planets that orbit stars other than our sun and exist outside our solar system. However, at this stage, a planet capable of supporting life was still out of the equation due to the hostile environments of the exoplanets discovered, and therefore, these findings didn't draw much attention from the public eye.
This was to change, though, as just a few years later, in 1995, the search for exoplanets around Sun-like stars that could support life bore fruit when Swiss astronomers Michel Mayor, then a professor at the University of Geneva, and his graduate student Didier Queloz made history. Using the radial velocity method, they detected a planet orbiting the star 1 Pegasi, a main-sequence star located about 50 light-years away in the constellation Pegasus. The planet, named 51 Pegasi b, is a gas giant similar to Jupiter, with 0.46 times its mass and 1.27 times its diameter. However, it orbits its star much more closely, completing an orbit in just 4.2 days.
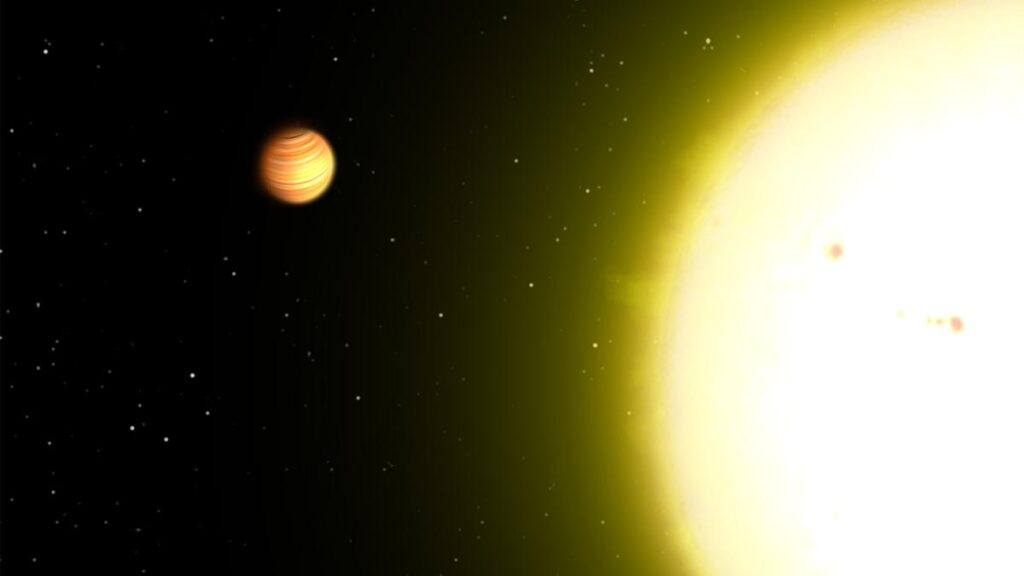
An artist's detailed impression of the gas giant exoplanet 51 Pegasi b, showcasing its close orbit around its parent star, 51 Pegasi, a G-type main-sequence star located approximately 50 light-years away in the constellation Pegasus. (Photo Credit: Wikimedia Commons)
Coming back, as there were skeptics, Queloz spent months convincing Mayor and the scientific community that the signal wasn’t an instrument error. This eventually led to their finding being corroborated by other astronomers, including Geoff Marcy and Paul Butler who were able to find the same signature in a different observatory. This discovery was revolutionary, as it confirmed that planets could exist around stars similar to our Sun, forever transforming our understanding of the universe. Mayor and Queloz were later awarded the 2019 Nobel Prize in Physics for their work, solidifying their legacy as pioneers in exoplanet research.
Discoveries & Methods
There are many ways to find exoplanets, with the radial velocity method, also known as the "Doppler wobble" technique, being the first. It involves detecting slight shifts in a star's spectral lines caused by the gravitational pull of an orbiting planet, which induces a wobble in the star's motion. This method has since been widely regarded as the first successful technique for detecting exoplanets, and it is ideal for ground-based telescopes because it does not require continuous monitoring of the stars. Now, in the 21st century came the Kepler Space Telescope, which was launched by NASA on March 6th, 2009, with the sole mission of searching for Earth-sized planets within the Milky Way but outside the Solar System. What method did it use, you ask? The specific method was the transit method, which observes transits—tiny dips in the brightness of a star when a planet crosses in front of it. During its first six weeks of operation, it was able to find five exoplanets: Kepler 4b, 5b, 6b, 7b, and, you guessed it, 8b. In April 2010 however, Kepler made its first major breakthrough, discovering the first confirmed planetary system with more than one planet transiting the same star, Kepler-9, after surveying over 156,000 stars for seven months. This was just the beginning.
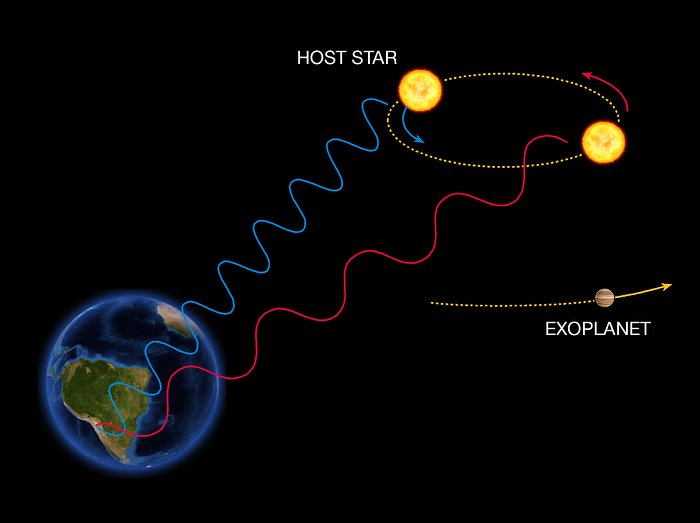
The radial velocity method detects exoplanets by observing variations in a star’s velocity caused by the gravitational pull of an orbiting planet. As the star moves toward us, its light is blueshifted; when it moves away, it is redshifted. By regularly measuring these shifts in the star’s spectrum, astronomers can determine if it is wobbling due to an unseen companion. (Photo Credit: ESO)
By February 2011, Kepler had uncovered a planetary system orbiting Kepler-11, a yellow dwarf star 2,000 light-years away, which contained six planets, all larger than Earth. These discoveries revealed that the most common planet size Kepler found didn’t exist in our solar system—a world between the size of Earth and Neptune. Additionally, Kepler identified densely packed planetary systems, some so tightly clustered around their parent stars that our own inner solar system appeared sparse by comparison. Kepler’s mission, which started with no knowledge of exoplanets outside our solar system, revealed that planets are everywhere, transforming our understanding of the universe. Despite challenges, including the failure of key reaction wheels in 2013, Kepler's mission continued through the K2 extension, surveying over 500,000 stars and collecting data on distant supernovae, black holes, and other celestial objects. Over the course of its mission, Kepler discovered nearly 3,000 new planets, further advancing the study of exoplanets. After nine years of invaluable data, Kepler was retired in 2018, leaving behind a legacy of over 2,600 confirmed planets and paving the way for future missions like NASA's Transiting Exoplanet Survey Satellite (TESS) and the James Webb Space Telescope to find more of the potential billions in the universe.
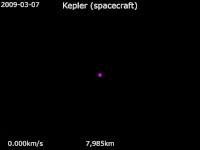
An animation depicting the trajectory of the Kepler space telescope from March 7, 2009, through its mission timeline, which is expected to conclude on December 31, 2060. The animation illustrates Kepler's orbit around Earth and its path as it gathers valuable data on exoplanets and distant star systems, contributing to our understanding of the universe over a span of more than five decades. (Photo Credit: NASA)
Now that we have understood the role of the Kepler Space Telescope in finding exoplanets, there is another method of finding them comes in the form of microlensing, a technique that allows astronomers to detect planets at truly great distances, far beyond the reach of other methods like radial velocity and transit photometry. While radial velocity surveys are limited to planets within around 100 light-years of Earth, and transit photometry can potentially detect planets within a few hundred light-years, microlensing can uncover planets orbiting stars near the center of the galaxy, thousands of light-years away. Microlensing occurs when the gravity of a foreground star bends the light from a more distant background star, creating a temporary increase in the brightness of the background star, as predicted by Einstein’s General Theory of Relativity. This bending of light is akin to the effect of a lens magnifying the distant star. If the foreground star has a planet, the planet’s gravity further distorts the light, creating a secondary brightening, or a “spike,” in the light curve, which can be detected as an additional fluctuation in the brightness. These spikes are the telltale signs of the presence of an exoplanet. Microlensing is particularly valuable because it can detect planets that are smaller in size, such as Earth-sized or even smaller planets, and those that orbit at larger distances from their parent stars—regions where other methods are less effective. It is also sensitive to free-floating or rogue planets that do not orbit any star at all. However, microlensing has its drawbacks. Since these events are rare and random, they are difficult to predict, and once the event has passed, the planet cannot be observed again. The distance of the planet from Earth can only be estimated roughly, and the data collected from a single microlensing event provides limited information about the planet, preventing further study of the same system. Despite these challenges, microlensing remains an essential tool in the quest to discover exoplanets, especially in the search for planets far from Earth, deep in the heart of our galaxy.
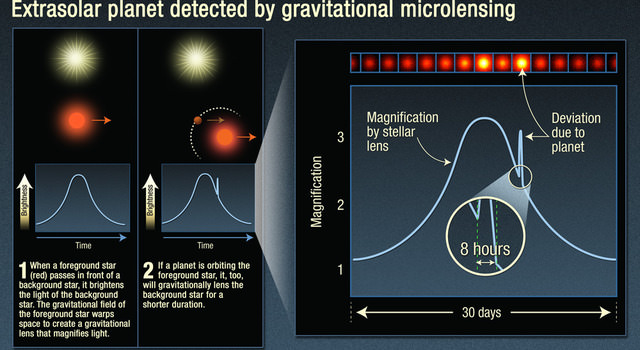
A detailed sketch illustrating the microlensing signature of a star system, with a planet positioned within the gravitational lensing system. (Photo Credit: NASA, ESA)
The last commonly known method is direct imaging, which allows astronomers to visually capture images of exoplanets. This method was notably demonstrated in November 2008 when astronomers using the Keck telescopes imaged three planets orbiting the star HR 8799. These planets, still retaining some heat from their formation, emit infrared radiation, making them visible even against the intense glare of their parent star. Unlike visible light, which is overpowered by the star's brilliance, the planets' infrared glow can be detected, allowing astronomers to observe their orbits. On the same day, astronomers using the Hubble Space Telescope images a planet orbiting the star Fomalhaut in visible light, aided by a thick disk of gas and dust surrounding the star. This disk helped scientists pinpoint the planet’s location, revealing its path through the debris. The planet, which appeared extraordinarily bright, was initially believed to be surrounded by a massive ring system, though it later vanished from view, leading astronomers to suggest it was a debris field from a planetary collision. As of 2020, over 50 exoplanets have been discovered through direct imaging, which provides valuable information about their properties, such as mass and temperature. This technique works best for planets that are far from their stars, where their light can be observed without interference from the star's glare. However, direct imaging is challenging due to the specific conditions required, making it unsuitable for large-scale exoplanet surveys. Future advancements in telescope technology, such as adaptive optics and coronagraphs, aim to improve direct imaging capabilities, potentially making it a more practical method for discovering exoplanets in a variety of star systems.
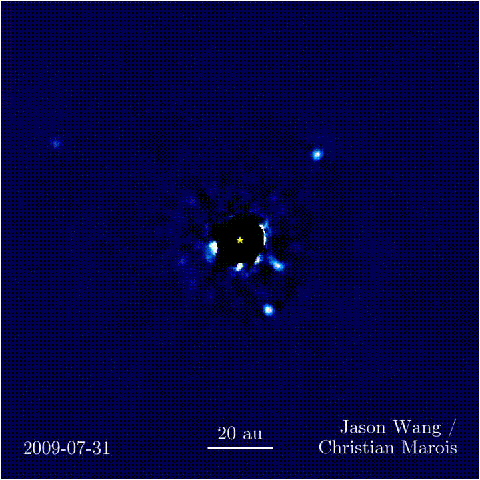
A direct image typically appears as shown here, with the star itself obscured in order to prevent its intense brightness from overwhelming the image. Without this adjustment, the light emitted by the star would be so powerful that it would obscure the finer details surrounding it, making it difficult to capture a clear and balanced representation of the surrounding space or objects. (Photo Credit: Jason Wang)
Exoplanet Types and Varieties
Exoplanets exhibit remarkable diversity, coming in a range of types that often defy the planetary configurations within our own solar system. These categories, each unique in their formation, structure, and atmospheres, provide insight into the vast and complex possibilities for planetary development throughout the galaxy.
One primary class of exoplanets includes gas giants, which are similar in composition and size to Jupiter and Saturn in our solar system. However, many of these planets are significantly larger or exhibit characteristics that challenge our solar system norms. Gas giants, which are primarily composed of hydrogen and helium, often have a relatively small rocky core surrounded by thick atmospheres that extend far into space. A major subset of gas giants are "Hot Jupiters," which orbit exceptionally close to their parent stars. These exoplanets maintain extremely high surface temperatures due to their proximity to their stars, causing their atmospheres to swell and expand. The intense heat can lead to strikingly different atmospheric conditions compared to those found on Jupiter or Saturn, such as extreme wind speeds and the presence of exotic gases not typically seen in colder, more distant planets. An example of a hot Jupiter is Kepler-39b, which orbits its star in just 2.5 days, resulting in an inflated atmosphere and a highly energetic environment. Similarly, HD 209458b, another hot Jupiter, has been the subject of detailed studies, with observations revealing atmospheric loss due to the extreme temperatures, a phenomenon that is more common among these close-orbiting giants.
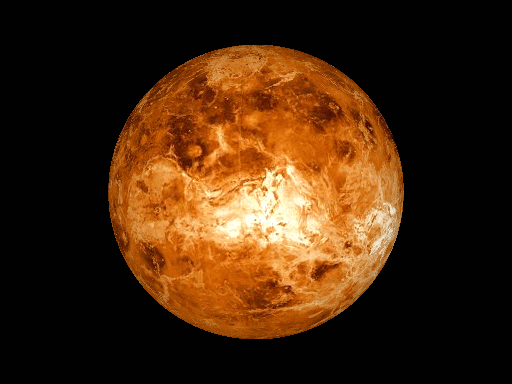
Gas giant exoplanets are planets that are made of gas and orbit stars outside of our solar system. They are easier to find than solid or terrestrial planets because of their large size that resembles known planets like Jupiter and Saturn. (Image credits: NASA)
Another notable subset of gas giants are "Cold Jupiters," which exist at much farther distances from their stars. These planets retain cooler temperatures and denser atmospheres that are more akin to the gas giants in our solar system. Cold Jupiters are often found in orbits similar to that of Jupiter and Saturn, with relatively stable and less extreme conditions. These planets also tend to have a more structured and layered atmosphere, with well-defined cloud bands and storm systems, similar to what we observe on Jupiter. An example of a cold Jupiter is HR 8799, a gas giant located in a system about 129 light-years away from Earth. It has been studied extensively because of its distance from its host star, which allows for more stable atmospheric conditions and easier observation. Other examples of cold Jupiters include Kepler-6b and Kepler-7b, both of which are located far from their stars and exhibit cooler atmospheres. Beyond cold and hot Jupiters, there are even larger categories of gas giants, such as "Super Jupiters," which are much larger in size and mass than Jupiter itself. These exoplanets, like the gas giants Kepler-22b and KELT-9b, often provide crucial insights into planetary formation and the effects of varying distance and mass on planetary environments. The diverse subcategories of gas giants illustrate the complexity and vast range of exoplanets in our galaxy, where factors such as distance from the host star, atmospheric composition, and size can lead to dramatically different planetary environments.
A second category of exoplanets, known as Neptunian Planets, refers to planets that are similar in size and composition to Neptune and Uranus. These planets typically have a rocky or icy core, surrounded by a thick atmosphere primarily composed of hydrogen, helium, and trace amounts of other gases, such as methane. Neptunian planets are characterized by their intermediate size, which places them between smaller terrestrial planets and large gas giants like Jupiter and Saturn. In many planetary systems, these planets are found closer to their stars in compact orbits, often within what is known as the "Neptunian desert"—a region where planets of similar size are notably absent. However, Neptunian planets often occupy a middle ground between rocky planets and gas giants, leading them to be a class of exoplanets that doesn't have a direct counterpart in our solar system. These planets are relatively common and make up a significant portion of known exoplanets discovered by missions like NASA's Kepler Space Telescope. Their size, ranging from approximately 1.5 to 4 times the radius of Earth, fills a gap between Earth-like planets and gas giants, representing a new and intriguing class of objects that broadens our understanding of planetary formation.
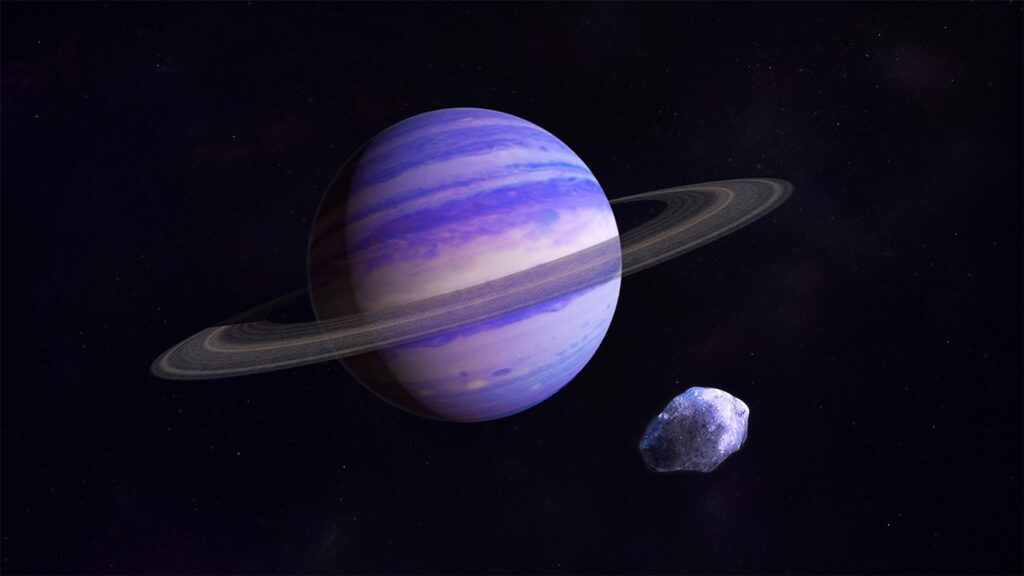
Neptunian exoplanets are similar in size to Neptune or Uranus and typically have hydrogen and helium-dominated atmospheres with cores of rock and heavier metals. (Image credits: NASA)
Neptunian planets often possess atmospheres that are quite different from those found on Earth, and this has led to some significant discoveries about their evolution. Their atmospheres can contain varying proportions of hydrogen, helium, and methane, though some may experience atmospheric loss due to intense stellar radiation, a process known as photoevaporation. This leads to the formation of smaller, more compact planets, sometimes referred to as "super-Earths." Recent studies, including data from the Kepler mission and ground-based telescopes, have shown that Neptunian planets are often found in a region between smaller, rocky planets and larger gas giants, which has sparked new theories about planetary migration. This process suggests that Neptunian planets may be formed at greater distances from their stars, only to later migrate inward to their current positions. As they approach their parent stars, these planets can undergo atmospheric stripping and other dynamic processes, which dramatically influence their structure and composition. This phenomenon has been particularly evident in "hot-Neptunes" that orbit very close to their stars, where radiation and high temperatures play a crucial role in shaping their evolution. The study of Neptunian planets, especially their migration patterns, provides astronomers with important clues about the formation and evolution of planetary systems, helping us better understand the diversity of planets beyond our solar system.
The category of Super-Earths represents one of the most fascinating and diverse classes of exoplanets discovered. Super-Earths are larger than Earth but smaller than Neptune, a size range that appears to be quite common among exoplanets despite lacking a direct analogue in our own planetary neighborhood. These planets can possess both rocky and gaseous characteristics, with atmospheres and surface compositions that vary widely depending on their distance from their star. Super-Earths are often found in tightly packed, multi-planet systems, showcasing a diversity that could potentially include habitable conditions. Their size range, which typically spans from about 1.5 to 10 times the mass of Earth, places them in a unique position to support a variety of geological and atmospheric conditions, from terrestrial, rocky surfaces to thick, gaseous envelopes. One of the intriguing aspects of Super-Earths is their potential for habitability. While many are located in what we call the "habitable zone" — the region around a star where liquid water could exist on the planet’s surface — their exact conditions depend on their atmospheric composition, which could include water vapor, carbon dioxide, and other gases. Some Super-Earths may even possess environments with dense atmospheres capable of trapping heat, creating "runaway greenhouse" effects similar to Venus. These planets can also host vast oceans or ice-covered surfaces, leading to various speculative models on their potential for supporting life. As we continue to discover and study Super-Earths, we not only learn more about the composition of exoplanets but also gain insight into the broader mechanics of planetary systems and the conditions that make a planet suitable for life.

An artist's depiction of exoplanet 55 Cancri e, seen here orbiting its star with its thick atmosphere, was found by scientists using data from the James Webb Telescope. (Image credits: JPL)
Finally, Terrestrial Planets, often referred to as "rocky planets," share many similarities with Earth in terms of their composition and internal structure, making them one of the most intriguing categories of exoplanets. These planets are typically composed of rock and metal, with a solid surface that can support a variety of geological processes. Terrestrial exoplanets are of particular interest in the search for habitable worlds, as they resemble Earth and Mars in structure and atmosphere, presenting the potential for environments conducive to life. Their geological features are diverse and may include evidence of past or present volcanic activity, tectonic movement, and the presence of mountains, valleys, and craters, all of which are characteristic of dynamic planetary surfaces.
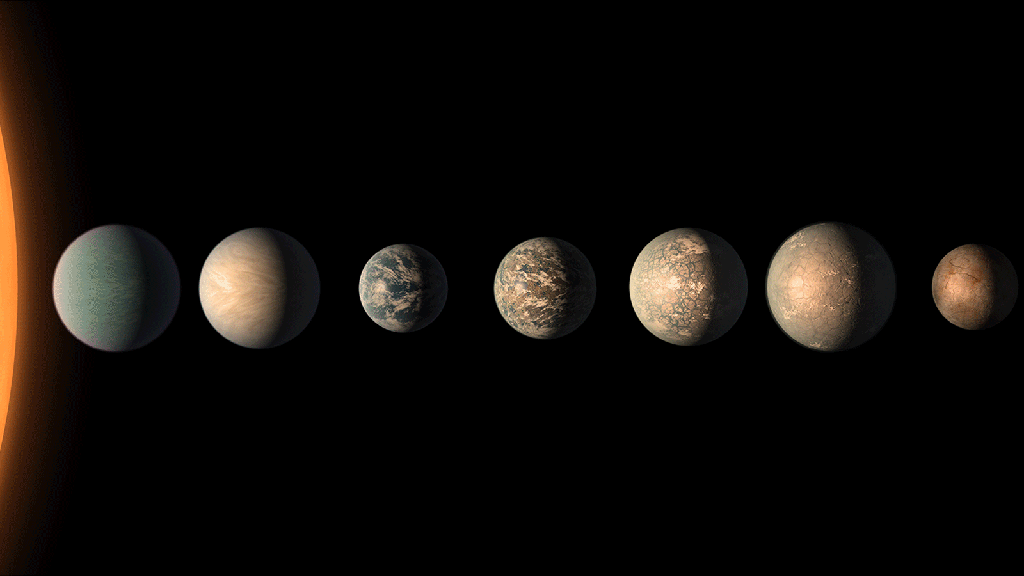
This artist's concept illustrates what the TRAPPIST-1 planets might look like, based on the available data regarding their diameters, masses, and distances from the host star, as of February 2018. (Image credits: NASA Science)
One of the most exciting aspects of terrestrial exoplanets is their potential to support liquid water, an essential ingredient for life as we know it. Many of these planets are located in the "habitable zone" around their stars, where temperatures are suitable for liquid water to exist on their surfaces. Examples of terrestrial exoplanets that have captured the attention of scientists include Kepler-452b, often referred to as "Earth’s cousin," which lies in the habitable zone of a star similar to the Sun. Another notable example is Proxima Centauri b, which orbits the closest star to our solar system, Proxima Centauri, and is located in its star's habitable zone. Under the right atmospheric conditions, these planets could sustain oceans, lakes, or rivers, similar to Earth’s own water cycle. Additionally, some terrestrial exoplanets may have atmospheres that provide a stable climate, offering a balance between greenhouse gases and other elements necessary for life. Scientists are particularly focused on finding rocky exoplanets that have conditions similar to early Earth, as they might provide insights into how life could emerge or thrive on other worlds.
Habitability and the Goldilocks Zone
A significant factor in determining a planet’s potential for supporting life is its location within the Goldilocks Zone—the region around a star where conditions are just right for liquid water to exist. This zone varies in size depending on the type of star. Around stars similar to our Sun, the Goldilocks Zone is typically located at a distance where the surface temperature of the planet can sustain liquid water, lying roughly between 0 and 100 degrees Celsius. For cooler stars, this habitable zone is much closer to the star, while for larger, hotter stars, the zone lies farther out.
However, recent studies suggest that habitability may not be strictly limited to planets within this zone. Planets located outside the Goldilocks Zone could still support liquid water if they possess dense atmospheres capable of retaining and redistributing heat. Such atmospheres might allow life-sustaining conditions even on planets that orbit closer to or farther from their stars than the traditional Goldilocks Zone would suggest. While no exoplanet has yet been confirmed to contain oceans or rivers, evidence of water molecules in the atmospheres of some exoplanets has been detected, hinting at the potential for habitable environments.
The first image of Hydra was downlinked from the New Horizons spacecraft on 15 July 2015, following its flyby. This image, taken from a distance of 640,000 km (400,000 mi), revealed brightness variations and a dark circular feature about 10 km (6.2 mi) across. The highest resolution image of Hydra which you can see above was captured from a distance of 231,000 km (144,000 mi), with a resolution of 1.2 km (0.75 mi) per pixel. Based on it, Hydra was estimated to have an approximate size of 55 km × 40 km (34 mi × 25 mi).
TrES-4b: The Largest Known Exoplanet
Discovered in 2007 by the Transatlantic Exoplanet Survey (TrES), TrES-4b is the largest known exoplanet by radius, about 1.8 times the size of Jupiter. Despite its enormous size, its mass is only about 0.84 times that of Jupiter, giving it a remarkably low density of 0.2 grams per cubic centimeter—lower than cork. This discovery challenged models of gas giant formation, as TrES-4b’s radius and low density imply a significant “puffiness” not well understood in the context of known gas giants. Its close orbit around its parent star, GSC 02620-00648, results in high surface temperatures exceeding 1,600 Kelvin, which is believed to contribute to its inflated atmosphere. The planet completes an orbit in just 3.6 Earth days, making it a quintessential "Hot Jupiter."
The extremely low density of TrES-4b remains a subject of study, with theories suggesting that high stellar radiation and possible atmospheric composition, including an abundance of lighter gasses like hydrogen and helium, contribute to its inflated state. Continued observations using spectroscopy are focused on detecting potential molecular compounds that could further elucidate this planetary anomaly.
Proxima Centauri b: The Closest Exoplanet to Earth
Proxima Centauri b, located just 4.24 light-years away, is part of the Alpha Centauri star system and orbits Proxima Centauri, the closest star to the Sun. Discovered in 2016 through the radial velocity method, Proxima b has a minimum mass of 1.17 Earth masses, suggesting that it may be rocky and potentially terrestrial. The planet orbits within its star's habitable zone at a distance of approximately 0.05 astronomical units, where conditions could theoretically allow for liquid water.
Despite its proximity and potential habitability, Proxima b faces challenges due to its orbit around a red dwarf star, which is prone to intense solar flares. These flares could strip away the atmosphere, posing a significant barrier to the planet’s habitability. Studies using spectral analysis aim to detect any atmosphere that may shield Proxima b’s surface from these harmful emissions. Additionally, upcoming missions, such as the Breakthrough Starshot initiative, aim to further investigate Proxima b through nano-probes, potentially providing the first close-up data on an exoplanet.
Kepler-186f: An Earth-Sized Planet in the Habitable Zone
Discovered by NASA's Kepler Space Telescope in 2014, Kepler-186f was the first Earth-sized exoplanet found within the habitable zone of its star, where temperatures could permit liquid water on its surface. Located about 500 light-years away in the constellation Cygnus, Kepler-186f orbits a red dwarf star at a distance that places it in a region with a moderate level of solar radiation. This exoplanet is only about 10% larger than Earth, making it one of the most Earth-like exoplanets discovered to date.
The discovery of Kepler-186f was groundbreaking as it demonstrated the existence of potentially habitable, Earth-sized planets around stars vastly different from our Sun. Although its red dwarf star emits less visible light, scientists theorize that photosynthesis might still be possible with infrared light. However, due to the limitations of current telescope technology, the planet’s atmospheric composition and surface conditions remain unknown. Scientists hope that the James Webb Space Telescope (JWST) will offer a more detailed look, potentially revealing atmospheric markers like oxygen or methane.
K2-18b: A Super-Earth with Potential Water Vapor
K2-18b, discovered by the K2 mission extension of the Kepler Space Telescope, orbits a red dwarf star about 120 light-years away in the constellation Leo. With a mass nearly eight times that of Earth, K2-18b falls into the “super-Earth” category, and its atmosphere likely contains water vapor, as detected in 2019 by the Hubble Space Telescope. While K2-18b lies within the habitable zone, making liquid water theoretically possible, its atmospheric pressure and composition are still under investigation.
The detection of water vapor on K2-18b was groundbreaking, marking the first such discovery for a planet in its star’s habitable zone. However, scientists debate whether K2-18b has a surface similar to Earth’s or is a “water world” with a deep ocean and dense atmosphere. The JWST and future telescopes aim to analyze the atmospheric layers of K2-18b, searching for biosignature gasses like ammonia or methane, which could indicate biological processes.
WASP-12b: A Planet Being Devoured by Its Star
WASP-12b, discovered in 2008, is a gas giant with a close orbit around its parent star, resulting in a rapid 1.1-day orbit and surface temperatures around 2,500 degrees Celsius. Located about 870 light-years away, this “Hot Jupiter” is notable for its elliptical, football-like shape caused by intense tidal forces from its host star. Spectroscopic studies reveal that WASP-12b’s atmosphere is slowly being stripped away by the star’s gravity, and it is expected to be engulfed by the star within the next 10 million years.
The case of WASP-12b provides a brief glance into the lifecycle of planets with close orbits, highlighting the fate that can await such gas giants as they fall under the gravitational influence of their stars. Observing WASP-12b offers insights into planetary disintegration and stellar dynamics, as well as the complex interactions between a star and its planet’s atmosphere.
Life Search and Detection Methods
The search for life on exoplanets has become one of the most compelling fields in modern astronomy, as scientists aim to answer whether Earth is unique in harboring life. Identifying life beyond our solar system necessitates specialized methods to detect potential biosignatures—chemical indicators of life—within exoplanetary atmospheres. The following section provides a detailed overview of the current approaches to searching for life on exoplanets, including the detection methods, tools, and promising techniques developed to identify potentially habitable environments and biosignatures.
Atmospheric Spectroscopy
One of the primary methods for detecting signs of life is atmospheric spectroscopy. This approach relies on analyzing the light that passes through or is reflected off an exoplanet’s atmosphere, which can reveal the composition of atmospheric gasses. When starlight interacts with a planet’s atmosphere during a transit (when the planet passes in front of its star), some wavelengths of light are absorbed by molecules in the atmosphere. By analyzing these absorption patterns, scientists can infer the presence of specific gasses.
Key Instruments: The Hubble Space Telescope (HST) and James Webb Space Telescope (JWST) are essential tools for conducting atmospheric spectroscopy. The HST has detected elements like water vapor, methane, and carbon dioxide on a few exoplanets. The JWST, with its advanced infrared capabilities, will provide higher-resolution spectra, allowing scientists to identify even trace amounts of molecules, including potential biosignatures such as oxygen, ozone, and methane.
Significance of Biosignature Gases: Certain gasses, particularly when found in combination, suggest potential biological processes. For example:
Oxygen (O₂): While oxygen alone can be produced through non-biological processes, large quantities combined with methane could imply biological activity, as these gasses tend to react and neutralize each other over time.
Methane (CH₄): Methane has both biological and non-biological sources, but sustained levels of methane, especially alongside oxygen, may hint at biological production.
Ozone (O₃): As a product of oxygen, ozone could indicate a protective layer around a planet, similar to Earth’s ozone layer, potentially shielding life from harmful UV radiation.
Spectroscopy has already identified water vapor on several exoplanets, including K2-18b. In 2019, studies detected water vapor in K2-18b’s atmosphere, marking a significant milestone as it is within the habitable zone of its star. This detection raises the possibility of liquid water, a prerequisite for life as we know it.
Direct Imaging
Direct imaging captures images of exoplanets by blocking out the light from their host stars, allowing scientists to study planetary surfaces and atmospheres. Although direct imaging is challenging due to the overwhelming brightness of stars compared to planets, advancements in telescope design have made it increasingly feasible.
Instruments and Techniques: Specialized instruments, such as the SPHERE (Spectro-Polarimetric High-contrast Exoplanet Research) instrument on the Very Large Telescope (VLT) and the Gemini Planet Imager (GPI), use techniques like coronagraphy and adaptive optics. Coronagraphs block the starlight to reveal dimmer planets nearby, while adaptive optics counteract atmospheric distortions.
Thus, Direct imaging allows astronomers to analyze an exoplanet’s surface conditions and atmospheric composition. For example, scientists are particularly interested in detecting reflective surfaces, such as ice caps, oceans, or land masses, which could suggest conditions similar to Earth. Additionally, imaging can reveal seasonal changes or atmospheric dynamics, giving further clues about the planet's environment and potential habitability.
One remarkable success story of direct imaging is the capture of the exoplanet HR 8799c, where astronomers directly observed clouds in its atmosphere. Although HR 8799c is a gas giant and not a candidate for life, this milestone proves the viability of imaging atmospheric features on distant planets.
Future Missions
The field of exoplanet life detection is set to advance significantly, driven by a new generation of missions and technologies designed to search for life beyond Earth. These initiatives aim to identify biosignatures—chemical indicators of life—in the atmospheres of distant planets, potentially transforming our understanding of habitable worlds and the likelihood of extraterrestrial life.
The James Webb Space Telescope (JWST), launched on December 25, 2021, is at the forefront of this effort. With its cutting-edge infrared capabilities, JWST is designed to study the atmospheres of exoplanets in unprecedented detail. It will focus on potentially habitable planets, analyzing molecules such as methane, carbon dioxide, and water vapor—key biosignatures that could indicate the presence of life. JWST will target systems like TRAPPIST-1, which hosts multiple Earth-sized planets within its habitable zone. Its observations, which began in 2022, are expected to provide crucial insights into the atmospheric conditions and potential habitability of these worlds.
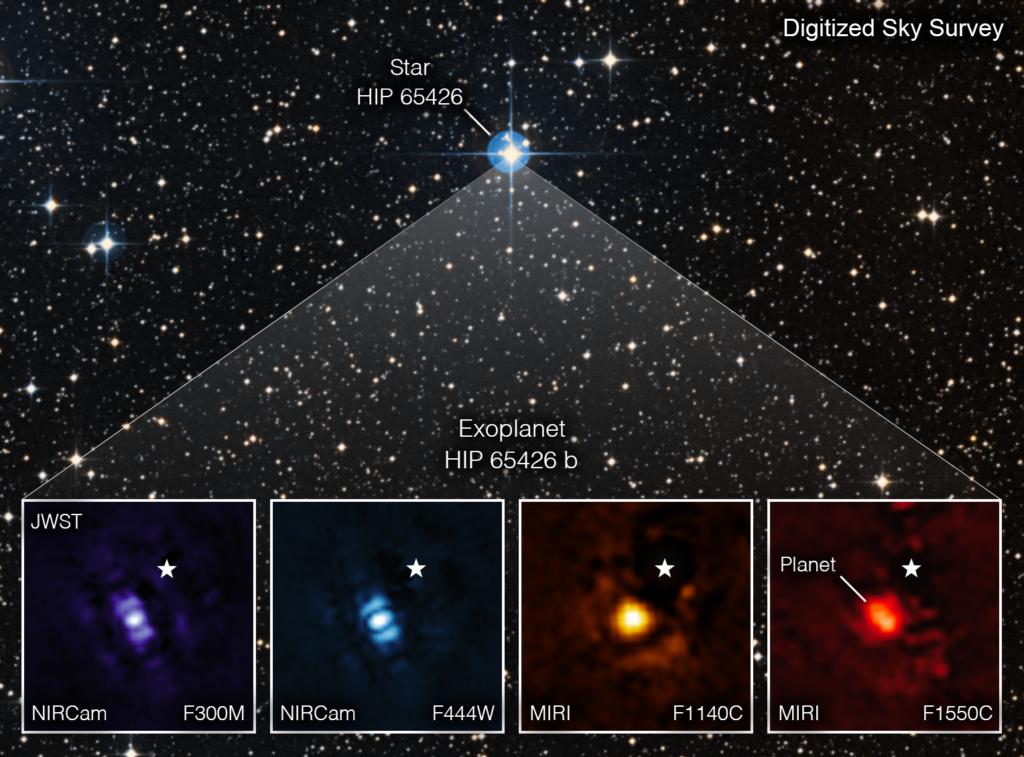
This image displays the exoplanet HIP 65426 b captured by the James Webb Space Telescope, showing it in various infrared light bands. The different colors represent different wavelengths: purple and blue from NIRCam at 3.00 and 4.44 microns, and yellow and red from MIRI at 11.4 and 15.5 microns. The white star shape indicates the location of the host star, HIP 65426, which has been removed using coronagraphs and image processing. (Image credits: JWST)
The Nancy Grace Roman Space Telescope, scheduled for launch in May 2027, will further advance our capabilities. Equipped with a high-contrast coronagraph, it will block out the overwhelming light from host stars, enabling direct imaging of Earth-like exoplanets. By capturing detailed images and analyzing the atmospheres of these distant worlds, the Roman Telescope will enhance our ability to detect biosignatures. It represents a critical step forward in the transition from indirect detection methods to direct atmospheric characterization.
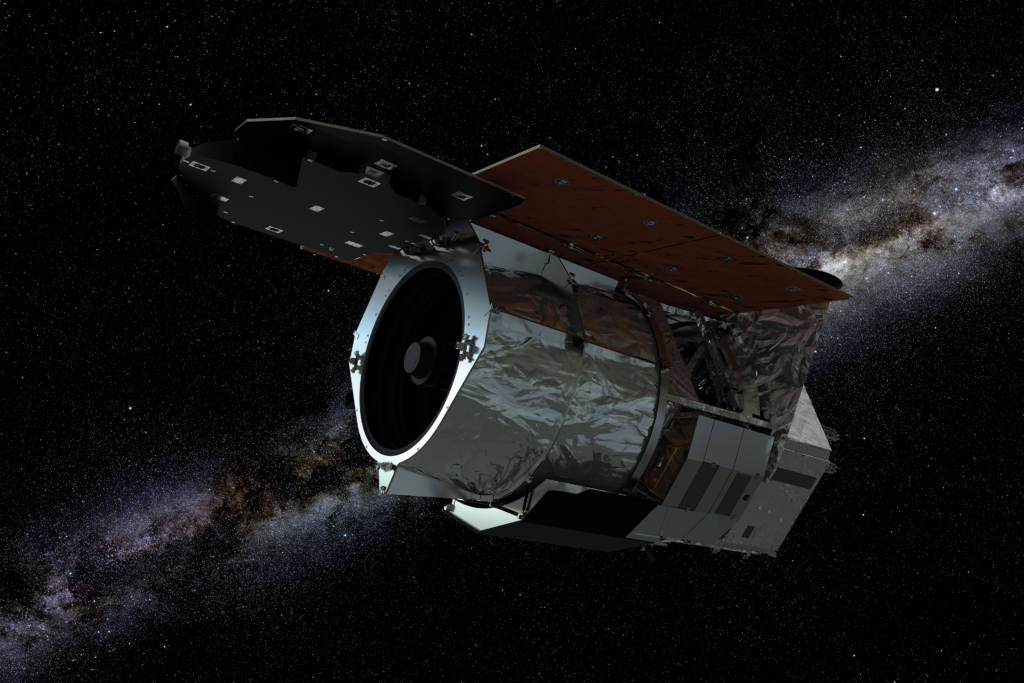
The image showcases the telescope's large primary mirror and sunshield, designed to observe distant galaxies, exoplanets, and other cosmic phenomena. With its advanced infrared capabilities, the Roman Space Telescope will contribute to our understanding of dark energy, exoplanet discovery, and the evolution of the universe. (Image credits: HubbleSite)
The Extremely Large Telescope (ELT), currently under construction in Chile, is expected to begin operations in 2028. With its massive 39-meter primary mirror, the ELT will be the largest optical and near-infrared telescope ever built. It will focus on small, rocky exoplanets around nearby stars, studying their atmospheres in search of biomarkers like oxygen and methane. The ELT’s advanced spectroscopic instruments will allow scientists to probe planets such as Proxima Centauri b, potentially providing the first direct evidence of biological activity beyond Earth.
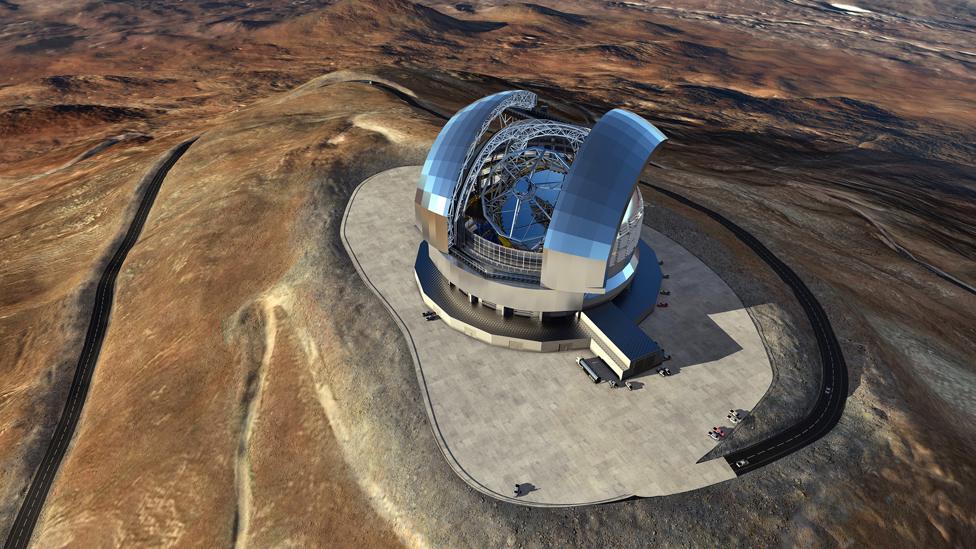
Located at Cerro Armazones in northern Chile, the 39-meter telescope is set to begin operations later this decade at the expense of 1.3 billion Euros. (Image credits: BBC)
Looking further ahead, NASA is developing two ambitious mission concepts: the Large UV/Optical/IR Surveyor (LUVOIR) and the Habitable Exoplanet Observatory (HabEx). These missions, proposed for launch in the 2030s or 2040s, aim to push the boundaries of exoplanet detection and characterization. LUVOIR, with a potential mirror size of up to 15 meters, and HabEx, featuring a starshade for optimal starlight suppression, will focus on Earth-sized exoplanets in habitable zones. By searching for a wide range of biosignatures, including ozone, nitrogen dioxide, and even industrial pollutants, these missions could extend the search for life to hundreds of exoplanets, offering unprecedented opportunities to detect worlds that might host life.
Conclusion
The study of exoplanets faces many challenges, but each discovery brings us closer to understanding the vast diversity and mystery of the universe—and to answering one of humanity’s oldest questions: Are we alone? Exoplanets not only deepen our comprehension of cosmic environments but also highlight the preciousness and rarity of our own world. With every new mission and technological breakthrough, the overwhelming silence of the cosmos lapses, capturing glimpses of distant worlds that may hold the ingredients for life or even serve as future homes for humanity. Since the first exoplanet discovery in the 1990s, our exploration has been driven by an enduring curiosity, a sense that somewhere, among untold billions of undiscovered worlds, life may exist.
As we embark deeper into our cosmic search, we carry with us the words of Arthur C. Clarke:
“Somewhere, something incredible is waiting to be known.”
Subscribe To Our Newsletter
Receive amazing space news and stories that are hot off the press and ready to be read by thousands of people all around the world.